The Role of Genomics in Clinical Research
Genomics, the study of an organism's complete set of DNA, including all of its genes, is a rapidly advancing field in modern science. It provides comprehensive insights into the genetic blueprint that governs biological functions and influences health and disease. The integration of genomic data into clinical research has opened new frontiers in understanding disease mechanisms, identifying novel therapeutic targets, and developing personalized medicine. This blog explores the transformative role of genomics in clinical research, highlighting its potential to revolutionize healthcare by offering more precise and effective treatments.
Clinical research is the systematic investigation of human health and diseases, aiming to improve diagnosis, treatment, and prevention. It encompasses various phases and types of studies, from basic laboratory research to large-scale clinical trials involving human participants. The primary goal of clinical research is to advance medical knowledge and translate scientific discoveries into practical applications that enhance patient care. The convergence of genomics and clinical research marks a new era in medicine, where the genetic basis of diseases can be studied in unprecedented detail, leading to more targeted and individualized approaches to treatment.
The purpose of this blog is to delve into the integration of genomic data in clinical research. We will explore the advances in genomic technologies, the challenges and solutions in managing and analyzing vast amounts of genetic data, and the exciting potential of genomics in identifying new drug targets and personalizing therapies. Additionally, we will discuss the challenges in bringing these advancements into clinical practice and the future directions in this rapidly evolving field.
Understanding Genomics and Clinical Research
Genomics encompasses the study of all aspects of an organism's genes and their functions. It goes beyond traditional genetics, which often focuses on individual genes, by considering the entire genome—the complete set of genetic material within an organism. This comprehensive approach allows researchers to understand complex biological systems, identify genetic variations associated with diseases, and explore how these variations influence health.
Clinical research, on the other hand, involves studies designed to test new treatments, interventions, or diagnostic methods in humans. It typically progresses through several phases: from early-phase trials that assess safety and dosage, to later-phase trials that evaluate efficacy and monitor side effects. Clinical research can take various forms, including observational studies, randomized controlled trials, and cohort studies. The integration of genomic information into these studies enhances the ability to identify genetic factors that contribute to disease, enabling the development of more effective and personalized medical interventions.
The intersection of genomics and clinical research heralds a new era of medicine—often referred to as precision medicine. Precision medicine aims to tailor healthcare to individual patients based on their genetic makeup, lifestyle, and environmental factors. This approach contrasts with the traditional one-size-fits-all model of medicine, which does not account for individual variability in disease risk and treatment response. By leveraging genomic data, researchers can develop more accurate diagnostic tools, identify new therapeutic targets, and design treatments that are more likely to be effective for specific patient subgroups.
Genomic Data Integration in Clinical Research
Advances in Genomic Technologies
The integration of genomics into clinical research has been propelled by significant advances in genomic technologies. One of the most transformative technologies is Next-Generation Sequencing (NGS), which allows for the rapid sequencing of entire genomes or targeted regions of DNA. NGS has dramatically reduced the cost and time required for sequencing, making it feasible to conduct large-scale genomic studies. This technology has been instrumental in identifying genetic mutations linked to diseases, understanding the genetic diversity within populations, and uncovering the genetic basis of rare and complex diseases.
Another groundbreaking technology is CRISPR-Cas9, a powerful tool for genome editing. CRISPR enables precise modifications of the DNA sequence, allowing researchers to study gene function, model diseases, and explore potential therapeutic applications. CRISPR has been used to correct genetic mutations in cell lines and animal models, providing insights into the underlying mechanisms of genetic disorders and opening up possibilities for gene therapy in humans.
Bioinformatics and computational biology are also essential components of genomic research. These fields involve the development and application of software tools to manage, analyze, and interpret vast amounts of genomic data. Advances in bioinformatics have enabled the identification of genetic variants associated with diseases, the prediction of protein structure and function, and the exploration of gene-environment interactions. Computational methods also play a crucial role in integrating genomic data with other types of biological data, such as transcriptomics and proteomics, to provide a more comprehensive understanding of biological processes.
Collection and Management of Genomic Data
The collection and management of genomic data are critical aspects of integrating genomics into clinical research. Sample collection typically involves obtaining biological specimens, such as blood, saliva, or tissue, from patients. These samples are then processed to extract DNA, which is sequenced and analyzed. The quality and integrity of the samples are vital for accurate genomic analysis, making proper handling and storage essential.
Managing the vast amounts of data generated by genomic studies presents significant challenges. Genomic data are not only large in volume but also complex, requiring sophisticated storage solutions and analytical tools. Data management systems must be able to handle the storage, retrieval, and analysis of data from diverse sources, including sequencing data, clinical records, and environmental information. Ensuring data security and privacy is also a major concern, as genomic data can reveal sensitive information about individuals and their families.
Ethical considerations are paramount in the handling of genomic data. Issues such as informed consent, data ownership, and the potential for genetic discrimination must be carefully addressed. Researchers must ensure that participants are fully informed about the use of their genetic information and that data is handled in a way that respects their privacy and autonomy. The establishment of ethical guidelines and regulatory frameworks is essential to protect the rights of participants and maintain public trust in genomic research.
Big Data Analytics in Genomics
The analysis of genomic data requires advanced computational techniques to process and interpret large datasets. Big data analytics in genomics involves the use of algorithms and statistical methods to identify patterns, associations, and causal relationships in genetic data. One of the key applications of big data analytics in genomics is the identification of genetic variants associated with diseases through genome-wide association studies (GWAS). These studies analyze the genomes of large groups of individuals to identify genetic markers that are more common in those with a particular disease compared to healthy individuals.
Artificial intelligence (AI) and machine learning are increasingly being used to analyze genomic data. These technologies can uncover complex patterns in data that are not apparent using traditional statistical methods. AI and machine learning algorithms can be used to predict disease risk, identify potential drug targets, and personalize treatment plans based on an individual's genetic profile. The use of AI in genomics is still in its early stages, but it holds great promise for advancing precision medicine.
Collaborative databases and data-sharing initiatives are crucial for advancing genomic research. Resources such as GenBank, the 1000 Genomes Project, and the Cancer Genome Atlas provide researchers with access to large-scale genomic datasets. These databases enable researchers to compare findings, validate results, and explore new hypotheses. Data sharing also facilitates international collaboration, allowing researchers from around the world to contribute to and benefit from the collective knowledge in the field.
Identifying Novel Targets for Drug Development
Understanding Disease Mechanisms
One of the primary benefits of integrating genomics into clinical research is the ability to understand the genetic basis of diseases. Genetic mutations can alter the function of proteins, disrupt cellular processes, and lead to the development of diseases. By studying these mutations, researchers can gain insights into the mechanisms underlying diseases and identify potential points of intervention. For example, mutations in the BRCA1 and BRCA2 genes are known to increase the risk of breast and ovarian cancers. Understanding these mutations has led to the development of targeted therapies and screening strategies for individuals with a high genetic risk.
Identifying biomarkers is another critical aspect of understanding disease mechanisms. Biomarkers are biological molecules that can be measured to indicate the presence or severity of a disease. Genomic biomarkers can include genetic mutations, gene expression patterns, or changes in DNA methylation. These biomarkers can be used for early diagnosis, prognosis, and monitoring of disease progression. For instance, the identification of specific genetic mutations in cancer cells can guide the selection of targeted therapies that are more likely to be effective for individual patients.
Target Discovery and Validation
The discovery and validation of new drug targets are essential steps in the drug development process. Genomics provides a wealth of information about the genes and proteins involved in disease processes, making it a valuable resource for identifying new therapeutic targets. Methods such as genome-wide association studies (GWAS) and functional genomics approaches can identify genes and pathways that play a critical role in disease. Once a potential target is identified, it must be validated through laboratory experiments and clinical studies to confirm its relevance to the disease and its potential as a therapeutic target.
Functional genomics involves studying the function of genes and their products to understand their role in disease. Techniques such as RNA interference (RNAi) and CRISPR-Cas9 can be used to silence or modify specific genes, allowing researchers to study the effects on cellular processes and disease outcomes. These studies can reveal the importance of specific genes in disease and identify potential targets for drug development. For example, CRISPR has been used to identify essential genes in cancer cells, providing new targets for cancer therapies.
Personalized Therapies: The Promise of Precision Medicine
Tailoring Treatments to Individual Genetic Profiles
Precision medicine aims to tailor medical treatments to individual patients based on their genetic profiles, lifestyle, and environmental factors. This approach contrasts with the traditional model of medicine, which often involves a one-size-fits-all treatment strategy. In precision medicine, genomic information is used to predict an individual's response to specific treatments, identify the most effective therapies, and minimize adverse effects. This personalized approach has the potential to improve treatment outcomes and reduce healthcare costs by avoiding ineffective treatments.
Pharmacogenomics is a key component of precision medicine, focusing on how genetic variations influence an individual's response to drugs. By understanding the genetic factors that affect drug metabolism, efficacy, and toxicity, healthcare providers can select the most appropriate medications and dosages for each patient. For example, certain genetic variants can affect the metabolism of warfarin, a commonly used anticoagulant, leading to variability in dosing requirements among patients. By genotyping patients before prescribing warfarin, clinicians can adjust the dose to minimize the risk of bleeding or clotting complications.
Personalized medicine has shown significant promise in oncology, where genetic testing can identify specific mutations in cancer cells that drive tumor growth. Targeted therapies, which are designed to interfere with these mutations, have been developed for various types of cancer. For instance, trastuzumab (Herceptin) is a targeted therapy used to treat HER2-positive breast cancer, a subtype characterized by the overexpression of the HER2 protein. By testing for HER2 overexpression, clinicians can identify patients who are likely to benefit from trastuzumab, improving treatment outcomes.
Case Studies and Success Stories
The application of genomics in clinical research and personalized medicine has led to several success stories, demonstrating its potential to improve patient outcomes. One notable example is the use of targeted therapies in treating chronic myeloid leukemia (CML). CML is often caused by the BCR-ABL fusion gene, which results from a chromosomal translocation. The discovery of this genetic abnormality led to the development of imatinib (Gleevec), a targeted therapy that inhibits the activity of the BCR-ABL protein. Imatinib has dramatically improved the prognosis for patients with CML, transforming it from a fatal disease to a manageable condition for many patients.
Another success story is the development of precision medicine approaches for cystic fibrosis, a genetic disorder caused by mutations in the CFTR gene. Ivacaftor (Kalydeco) is a drug that targets specific CFTR mutations, restoring the function of the defective protein. The approval of ivacaftor marked a significant milestone in the treatment of cystic fibrosis, as it was the first drug to address the underlying genetic cause of the disease. Subsequent development of combination therapies, such as lumacaftor/ivacaftor (Orkambi), has expanded the treatment options for patients with different CFTR mutations.
These success stories highlight the potential of personalized medicine to improve treatment outcomes by targeting the underlying genetic causes of disease. They also underscore the importance of ongoing research and innovation in the field of genomics and clinical research.
Challenges and Future Directions
Challenges in Integrating Genomics into Clinical Practice
Despite the significant advancements in genomics and personalized medicine, several challenges remain in integrating these approaches into routine clinical practice. One of the primary challenges is the technical and logistical complexity of genomic testing. Sequencing and analyzing an individual's genome requires specialized equipment, expertise, and resources. The interpretation of genomic data can also be challenging, as it involves distinguishing between benign genetic variations and those that are clinically significant.
Cost and accessibility are also major barriers to the widespread adoption of genomic testing. While the cost of sequencing has decreased significantly, it remains relatively high, particularly for whole-genome sequencing. Additionally, access to genomic testing and personalized medicine may be limited in resource-constrained settings, exacerbating health disparities. Ensuring equitable access to these technologies is essential for realizing the full potential of precision medicine.
Ethical and legal considerations are critical in the field of genomics. Issues such as informed consent, privacy, and the potential for genetic discrimination must be carefully addressed. The storage and sharing of genomic data raise concerns about data security and patient confidentiality. The establishment of robust ethical guidelines and regulatory frameworks is essential to protect the rights of patients and maintain public trust in genomic research.
Future Trends in Genomics and Clinical Research
Looking ahead, several emerging trends in genomics and clinical research hold promise for further advancing the field. Single-cell sequencing is an emerging technology that allows for the analysis of individual cells' genomes, transcriptomes, and epigenomes. This technology provides unprecedented insights into cellular heterogeneity and the complex interplay of genetic and environmental factors at the single-cell level. Single-cell sequencing has applications in cancer research, developmental biology, and immunology, among other fields.
The potential of gene therapies and CRISPR-based treatments is another exciting area of research. Gene therapies involve the introduction, removal, or alteration of genetic material within a patient's cells to treat or prevent disease. CRISPR-based gene editing offers a precise and efficient method for making genetic modifications. Several gene therapy and CRISPR-based treatments are currently in clinical trials, with promising results. These therapies have the potential to cure genetic disorders, such as sickle cell disease and hemophilia, and offer new treatment options for various diseases.
The growing role of artificial intelligence and machine learning in genomics is also expected to drive innovation in the field. AI and machine learning algorithms can analyze vast amounts of genomic data, identify patterns, and make predictions that can inform clinical decision-making. These technologies have the potential to improve the accuracy and efficiency of genomic analyses, enhance the discovery of new drug targets, and personalize treatment plans based on an individual's genetic profile.
Notable Labs: Exploring the Role of Genomics in Clinical Research
Genomics, the comprehensive study of an organism's complete set of DNA, has become a pivotal element in modern clinical research. It offers invaluable insights into the genetic underpinnings of diseases, paving the way for innovative therapeutic approaches and personalized medicine. Notable Labs is at the forefront of integrating genomic data into clinical research, aiming to revolutionize healthcare.
In this blog, we delve into the transformative role of genomics, exploring advances in technologies like Next-Generation Sequencing (NGS) and CRISPR-Cas9, which have drastically reduced the cost and time required for genomic analysis. These technologies enable the identification of genetic mutations associated with diseases and the development of targeted therapies tailored to individual genetic profiles. The integration of genomics in clinical trials and drug development processes promises more precise and effective treatments, ushering in a new era of precision medicine.
Notable Labs is committed to overcoming challenges in genomic data management and analysis, ensuring the ethical and equitable use of genetic information. As we look to the future, the potential of genomics in clinical research continues to expand, offering hope for more personalized and effective healthcare solutions.
Conclusion
In conclusion, the integration of genomics into clinical research is revolutionizing healthcare by providing new insights into the genetic basis of diseases and enabling the development of personalized therapies. Advances in genomic technologies, such as Next-Generation Sequencing and CRISPR, have facilitated the collection and analysis of vast amounts of genetic data. This information has been used to identify novel drug targets, develop targeted therapies, and tailor treatments to individual patients' genetic profiles.
While significant progress has been made, challenges remain in integrating genomics into routine clinical practice. Technical, logistical, and ethical issues must be addressed to ensure the equitable and responsible use of genomic data. Looking forward, emerging technologies, such as single-cell sequencing and gene therapies, hold promise for further advancing the field of genomics and precision medicine.
The transformative potential of genomics in clinical research and personalized medicine cannot be overstated. By continuing to invest in research, collaboration, and ethical considerations, we can harness the power of genomics to improve patient outcomes and usher in a new era of precision medicine.
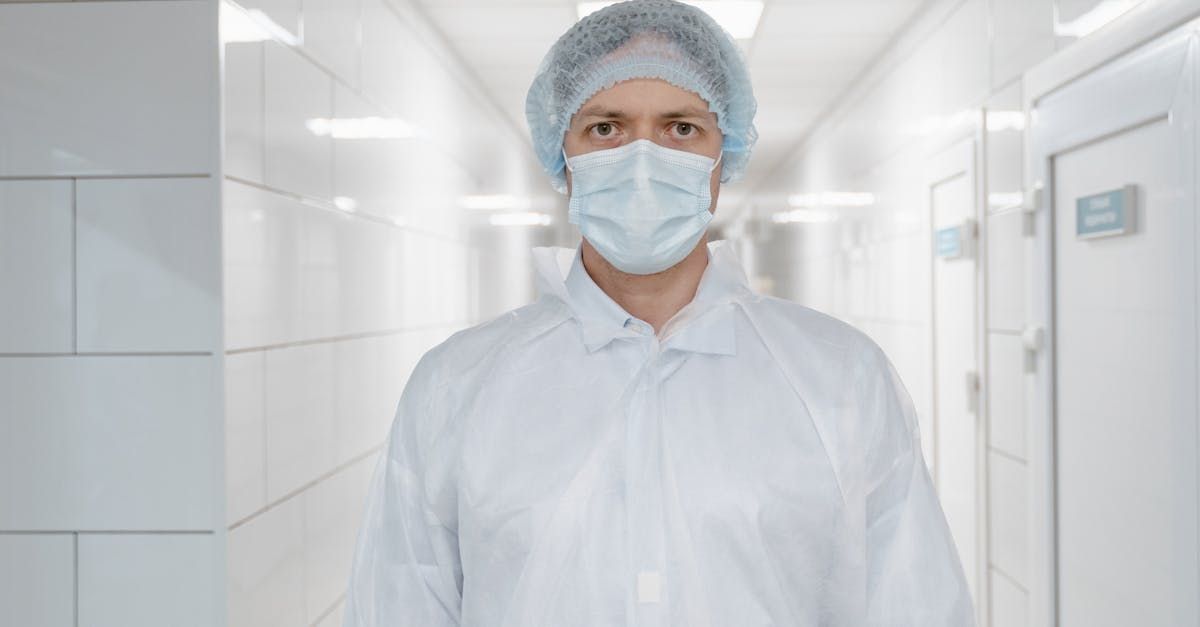
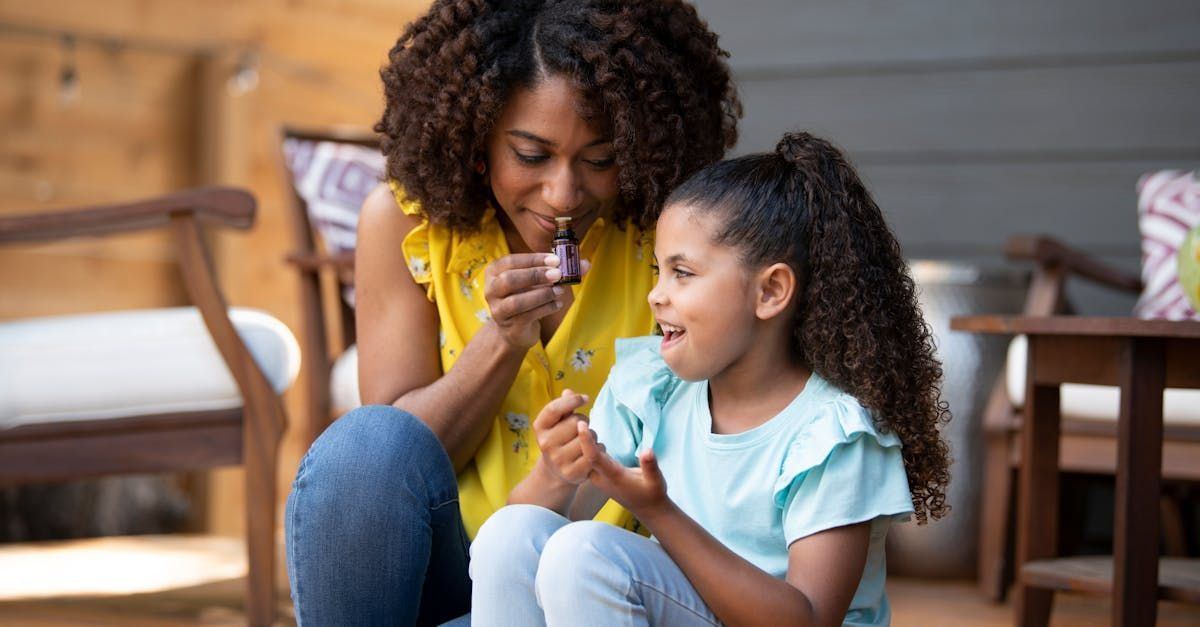
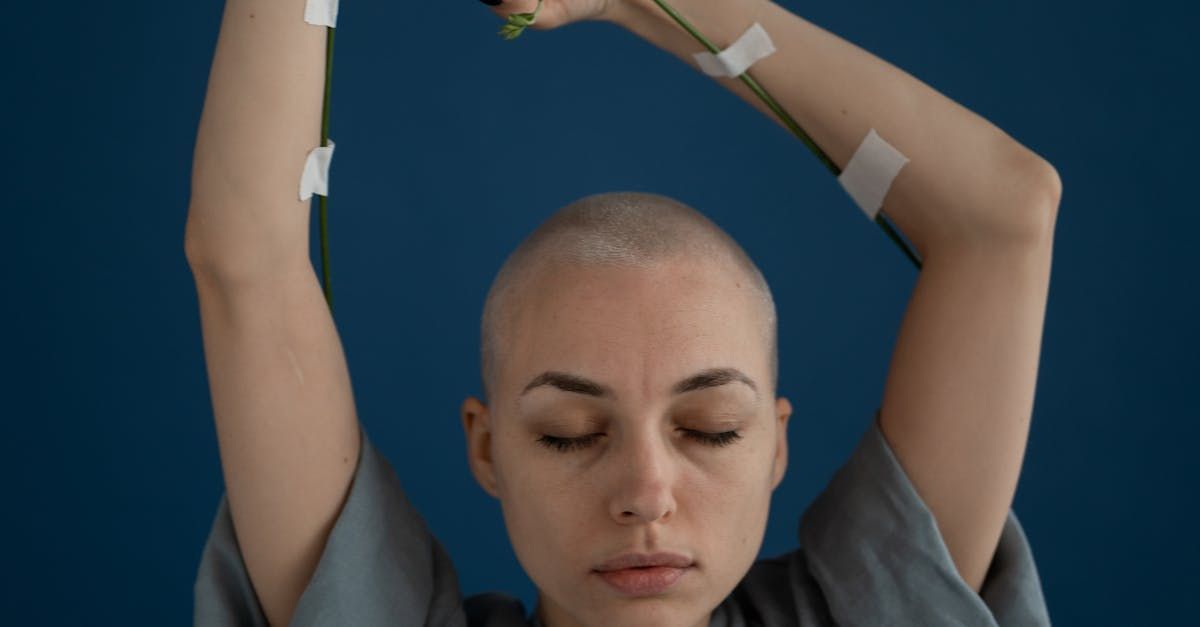
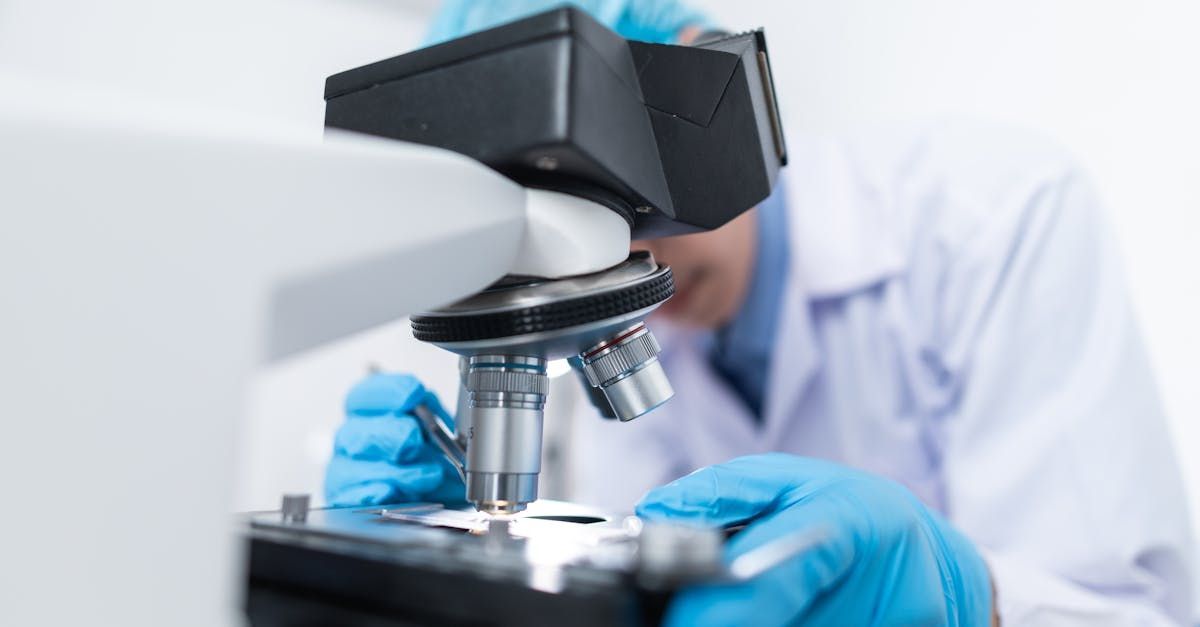
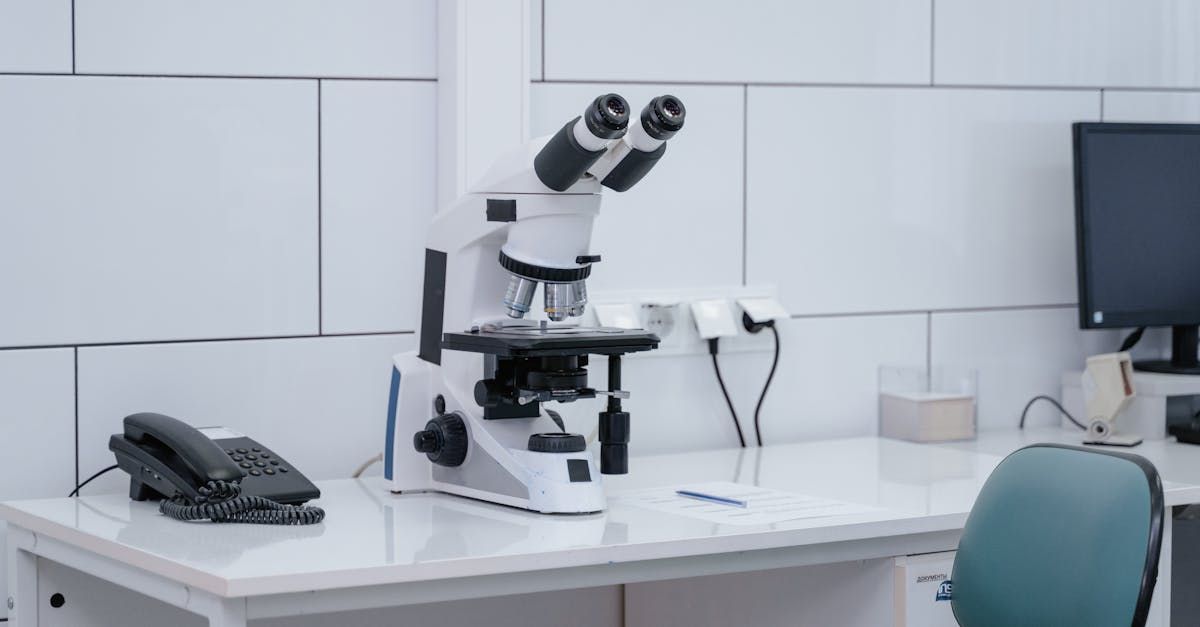
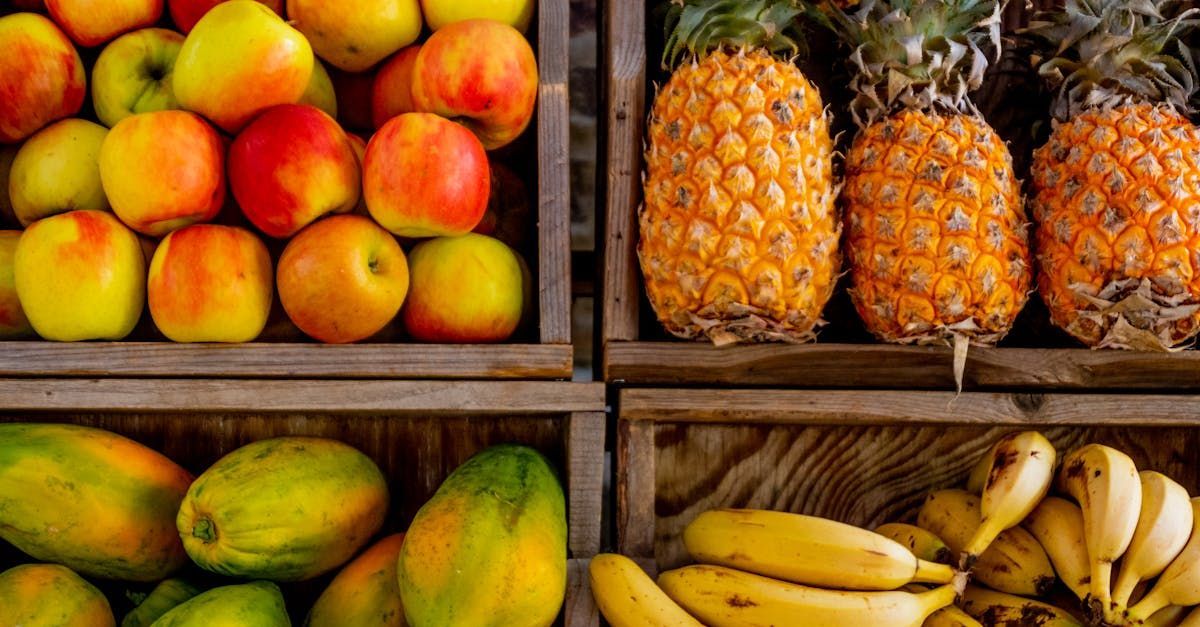
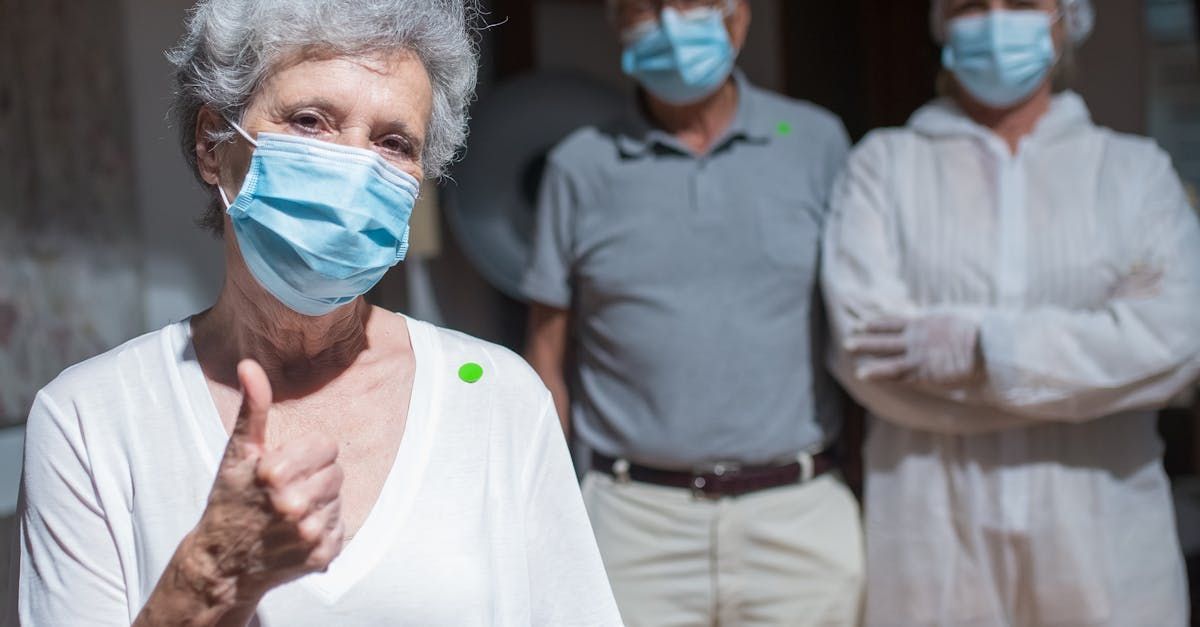
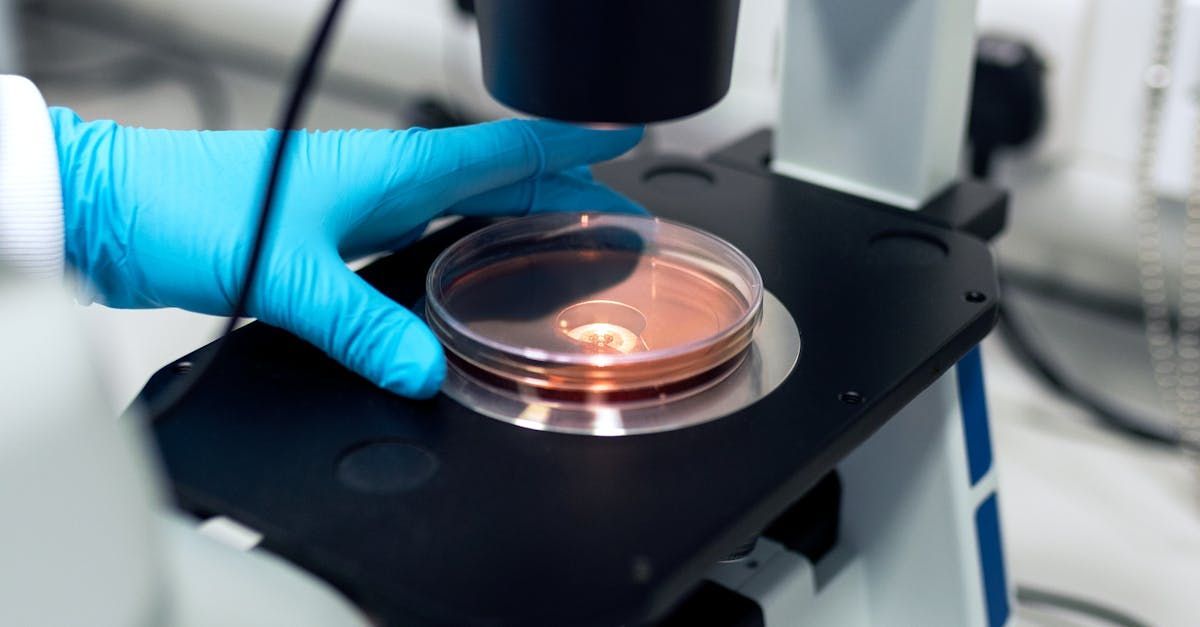
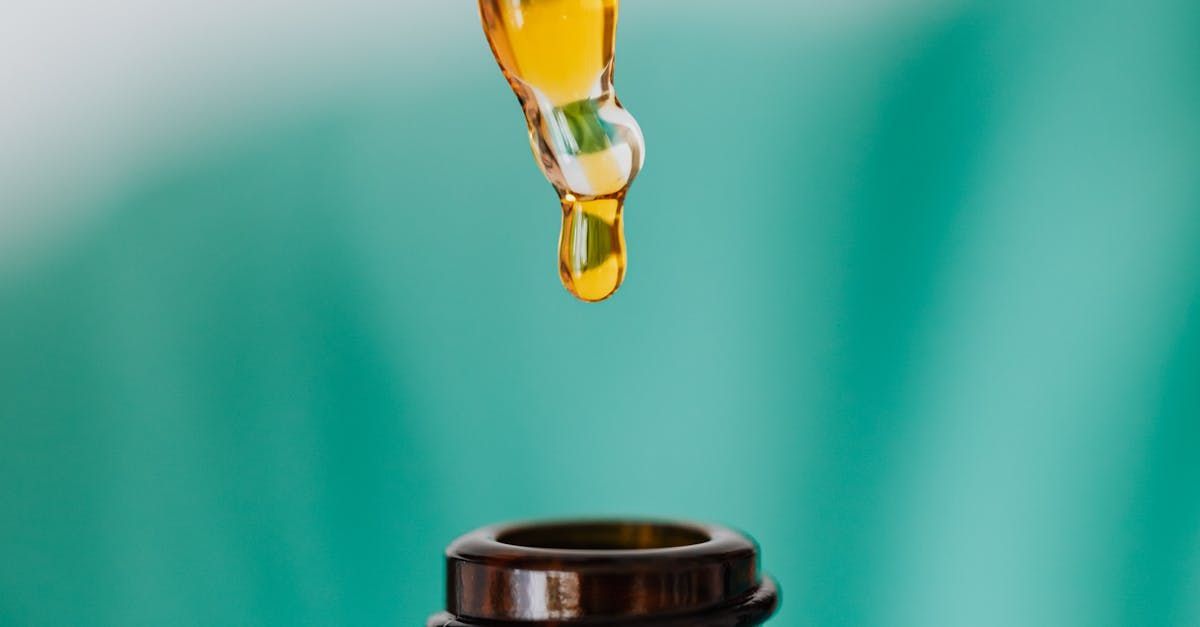
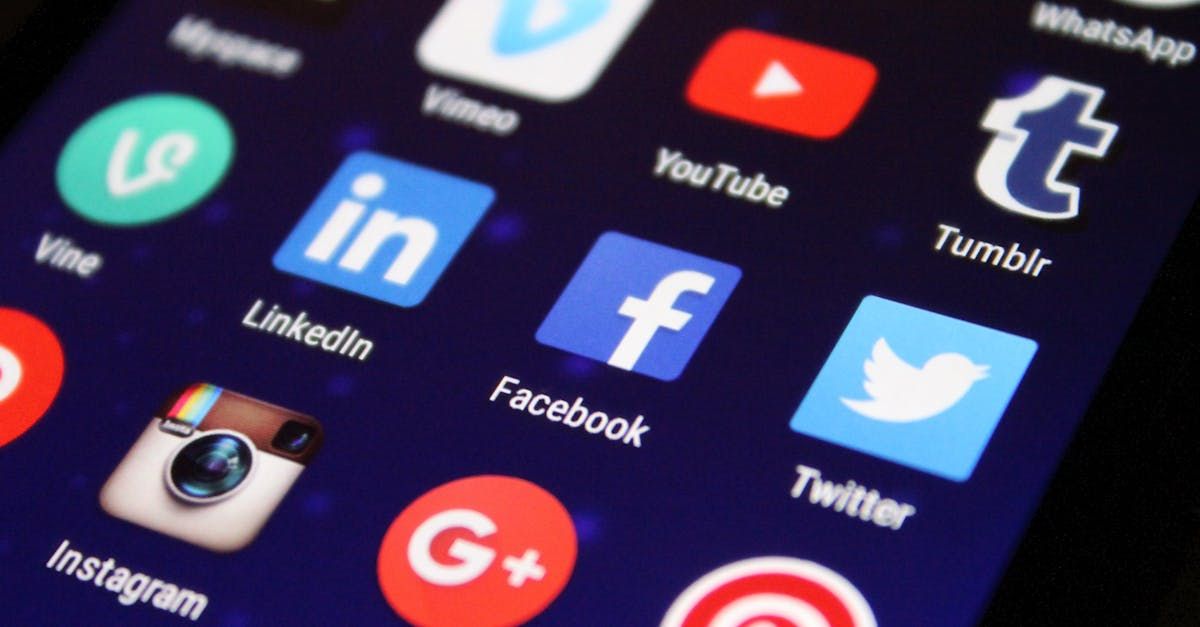